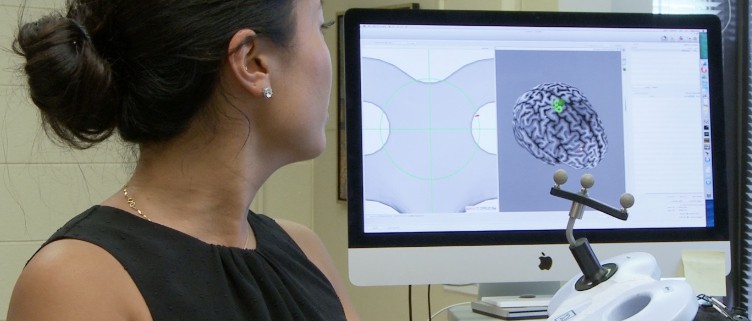
Prefrontal transcranial magnetic stimulation for depression in US military veterans – A naturalistic cohort study in the veterans health administration
Abstract
Background
Repetitive transcranial magnetic stimulation (TMS) is an evidence-based treatment for pharmacoresistant major depressive disorder (MDD), however, the evidence in veterans has been mixed. To this end, VA implemented a nationwide TMS program that included evaluating clinical outcomes within a naturalistic design. TMS was hypothesized to be safe and provide clinically meaningful reductions in MDD and posttraumatic stress disorder (PTSD) symptoms.
Methods
Inclusion criteria were MDD diagnosis and standard clinical TMS eligibility. Of the 770 patients enrolled between October 2017 and March 2020, 68.4% (n = 521) met threshold-level PTSD symptom criteria. Treatments generally used standard parameters (e.g., left dorsolateral prefrontal cortex, 120% motor threshold, 10 Hz, 3000 pulses/treatment). Adequate dose was operationally defined as 30 sessions. MDD and PTSD symptoms were measured using the 9-item patient health questionnaire (PHQ-9) and PTSD checklist for DSM-5 (PCL-5), respectively.
Results
Of the 770 who received at least one session, TMS was associated with clinically meaningful (Cohen’s d>1.0) and statistically significant (all p<.001) reductions in MDD and PTSD. Of the 340 veterans who received an adequate dose, MDD response and remission rates were 41.4% and 20%, respectively. In veterans with comorbid PTSD, 65.3% demonstrated clinically meaningful reduction and 46.1% no longer met PTSD threshold criteria after TMS. Side effects were consistent with the known safety profile of TMS.
Limitations
Include those inherent to retrospective observational cohort study in Veterans.
Conclusions
These multisite, large-scale data supports the effectiveness and safety of TMS for veterans with MDD and PTSD using standard clinical approaches.
Keywords
1. Introduction
Major depressive disorder (MDD) affects patients across the globe as a leading cause of disability (Friedrich, 2017), is a major contributor to suicide, and a risk factor for poor medical outcomes. Current treatments rely upon medications and psychotherapy, with limited options for the approximately one third of patients who do not respond (Rush et al., 2006). Other options typically include electroconvulsive therapy, which is limited in use due to risks of anesthesia and short-term memory impairment (Sackeim 2017). While there are investigations of emerging novel agents (e.g., (es)ketamine, psilocybin, etc.) (Davis et al., 2020; McIntyre et al., 2021) there are concerns about abuse potential and long-term effectiveness.
Repetitive transcranial magnetic stimulation (rTMS, hereafter TMS), an evidence-based treatment for pharmacoresistant MDD, has been cleared by the US Food and Drug Administration since 2008 (O’Reardon et al., 2007; George et al., 2010). Although there is a rare risk of seizure during stimulation, TMS has a favorable safety profile without systemic side effects (weight gain, diabetes, etc.). Data has now emerged supporting TMS across psychiatric disorders, including obsessive-compulsive disorder, smoking cessation, posttraumatic stress disorder (PTSD), and schizophrenia (reviewed in (George, 2019; Cosmo et al., 2021).
One patient population of interest is US military veterans. Several factors related to Veteran care are relevant to TMS. These patients have elevated rates of medical and psychiatric comorbidity (Agha et al., 2000) as well as suicide risk (2017). Given these challenges to treatment, veterans stand to benefit further from non-pharmacological treatments that may also reduce suicidality. Furthermore, TMS access and delivery are likely to be different in the VA, as private insurance is not involved in eligibility determination. Interestingly, unlike in the general population, the efficacy data on TMS in Veterans has been mixed. Unlike other TMS efficacy studies (O’Reardon et al., 2007; George et al., 2010), a recent controlled trial in veterans by Yesavage et al. (2018) found that active TMS did not separate from sham, and comorbid PTSD was associated with poorer outcomes. Interestingly, this study yielded high remission rates (defined as obtaining scores below threshold values on standardized rating scales) in both active (40.7%) and sham (37.4%) groups. While the reason for these results remains debated in the field, this study had strict monitoring of medication usage that reminded participants to take their daily psychiatric medications. This monitoring likely caused some participants to begin regularly taking their medication which resulted in effectively starting the medication and the TMS (active and sham) simultaneously. To this point, two other studies that failed to differentiate active from sham (Herwig et al., 2007; Brunelin et al., 2014) initiated antidepressants at the same time as TMS. Despite findings from Yesavage et al., other studies have consistently indicated efficacy of TMS for Veterans, including those with comorbid MDD and PTSD (Karsen et al., 2014; Kozel et al., 2019; Philip et al., 2019). In addition, an analysis of clinical outcomes of TMS for depressed veterans (n = 118; with nominal overlap with the current report) found PTSD did not impact MDD response or remission rates (Hernandez et al., 2020). Furthermore, a recent meta-analysis supports the efficacy of TMS for PTSD (Kan et al., 2020).
Within the context of these mixed data, and to address the health needs of veterans, the Veterans Health Administration (VA) instituted a nationwide multisite TMS program, informed by prior positive controlled trials (O’Reardon et al., 2007; George et al., 2010), and naturalistic studies (Carpenter et al., 2012). This program was designed to provide evidenced-based TMS for MDD to veterans and evaluate clinical effectiveness and safety. This report represents the first outcomes from the program. We hypothesized TMS would be safe and effective for MDD and comorbid PTSD. We also assessed patients who received an adequate TMS dose, operationalized as at least 30 daily sessions, and compared to the entire cohort.
2. Methods
2.1. Project organization
The VA Palo Alto Healthcare System Mental Illness Research Education and Clinical Center (MIRECC) served as the coordinating site for this retrospective observational cohort study. The VA Palo Alto/Stanford Institutional Review Board approved associated procedures as quality improvement and data was gathered via VA REDCap. The “VA TMS Pilot Program” distributed devices, identified existing TMS clinics, and provided high quality training (Carrico et al., 2020). The VA has a strong track record of implementing evidence-based care (e.g., Eftekhari et al. 2013), which can be harnessed to disseminate novel treatments and provide infrastructure for important research questions (Williams et al., 2021).
2.2. Referral and treatment information
Patients were referred by their primary mental healthcare provider, and determined to be eligible if they met TMS indication criteria (e.g., failure of >1 antidepressant in the current depressive episode). The recommendation was for patients to have a MDD diagnosis, which could be comorbid with other diagnoses such as PTSD. Standard contraindications were utilized (e.g., seizures, intracranial metal, etc.). A TMS-credentialed VA physician performed an initial evaluation and verified other treatments (e.g., medication, psychotherapy, etc.) were unchanged six weeks prior to stimulation. No medication was absolutely contraindicated, and treatment continued unchanged throughout TMS (the impact of medications will be the topic of a future report). Clinical factors, such as adherence or substance use, were assessed daily following standard clinical care.
2.3. Devices and parameters
TMS devices included the Magstim Super Rapid2, Horizon Lite, or Horizon Performance device (Wales, UK); some sites used others purchased outside the program (e.g., Neuronetics, Malvern PA, USA; MagVenture, Farum, Denmark; Brainsway, Jerusalem, Israel). Motor threshold determination was performed prior to stimulation and defined as the amount of energy applied to the motor cortex to elicit movement in the contralateral fingers (typically abductor pollicis brevis) 50% of the time. The TMS target was the left dorsolateral prefrontal cortex (DLPFC), located using the Beam/F3 method (Beam et al., 2009). Sites were advised to follow FDA guidelines, and generally administered high frequency stimulation, e.g., daily TMS delivered at 10 Hz to the left DLPFC at 120% of motor threshold for up to 30 sessions followed by a six-session taper. Based on clinical judgement, parameters could be modified when following an evidence-based off-label approach, which was reported in the REDCap database (Table 2).
2.4. Measures and statistical analyses
Standardized self-report rating scales were selected based on those used in clinical (non-research) settings to provide adequate information about symptom severity while minimizing burden. Primary outcomes were changes in MDD and PTSD symptom severity; subsequent reports will include other assessments. MDD and PTSD were measured using the 9-item patient health questionnaire (PHQ-9; (Kroenke et al., 2001)) and PTSD symptom checklist for DSM5 (PCL-5; (Weathers et al., 2013a)), respectively. Outcomes of interest included mean changes, dichotomous/categorical outcomes describing MDD clinical response (>50% reduction) and remission (score<5) on the PHQ9, and PTSD outcomes of clinically meaningful reduction (>10 points) and those no longer meeting threshold severity criteria for probable PTSD (operationally defined as score <33 on the PCL-5 (following (Weathers et al. (2013a)). Outcomes were analyzed using paired-sample t-tests for continuous outcomes, descriptive statistics for categorical outcomes, and analysis of variance to assess symptom changes over time. Patients were excluded from relevant analyses if baseline scores were less than remission (MDD) or clinically relevant PTSD severity (PTSD). Missing data were reconciled via site query, with individual sites reporting dropouts and associated reasons. Effect sizes (Cohen’s d Cohen, 1988) and confidence intervals were generated for continuous outcomes. Sensitivity analyses were performed on several key areas of clinical interest, including definition of adequate dose and TMS parameters. Safety was assessed via spontaneous patient report recorded in the database and site queries.
This report includes all veterans that received TMS at 27 VA sites from October 2017 until March 2020 (i.e., pre-COVID-19). Results were analyzed using two approaches; first, following modified “intent-to-treat” principles, where patients who received baseline assessments and >1 TMS session were included in the “entire group” analyses. This was followed by analyses focused on the “adequate dose group” who received an adequate dose of TMS, operationalized as at least 30 sessions (i.e., a single daily session for six weeks).
3. Results
3.1. PATIENT characteristics
Seven hundred seventy (n = 770) patients were in the entire group and 340 received an adequate dose. Demographics were consistent with veterans (i.e., majority white and male), yet many races, ethnicities, and genders were represented (Table 1). Military service encompassed multiple eras (e.g., Vietnam, Gulf War, Operation Iraqi and Enduring Freedom, etc.). There were no meaningful site differences in baseline symptom severity (all p >.1). For this examination, patients were followed for the duration of TMS (i.e., 6–8 weeks). The majority of patients received 10 Hz TMS to the left DLPFC (n = 595; 69.8%), with modest representation of other approaches (Table 2).
Table 1. Demographics and Clinical Characteristics.
Entire Groupa (n = 770) | Adequate Dosea (n = 340) | |
---|---|---|
Age (mean, SD) | 51.84 (14.11) | 53.39 (13.97) |
Race | ||
White | 552 (71.6%) | 260 (76.1%) |
Black | 88 (11.4%) | 39 (11.5%) |
Asian | 18 (2.3%) | 7 (2.2%) |
Multiracial | 16 (2.1%) | 4 (1.3%) |
Otherb | 49 (6.5%) | 15 (4.4%) |
No response | 47 (6.1%) | 15 (4.5%) |
Biological Sex | ||
Male | 592 (76.8%) | 269 (79%) |
Female | 141 (18.4%) | 60 (17.8%) |
No response | 37 (4.8%) | 11 (3.2%) |
Gender | ||
Male | 394 (51.2%) | 163 (47.8%) |
Female | 105 (13.8%) | 48 (14.0%) |
Transgender | 7 (0.9%) | – |
Androgynous | 1 (0.1%) | – |
Agender | 5 (0.6%) | 1 (0.3%) |
No response | 258 (33.5%) | 129 (37.9%) |
Highest Education Achieved | ||
Elementary School | 5 (0.6%) | 2 (0.6%) |
High School or GED | 72 (9.4%) | 22 (6.4%) |
Some college, no degree | 228 (29.6%) | 99 (29%) |
Associate degree, vocational program, or certificate | 121 (15.7%) | 54 (15.9%) |
Bachelor’s degree | 144 (18.7%) | 67 (19.7%) |
Master’s degree | 88 (11.4%) | 45 (13.1%) |
Doctoral degree | 20 (2.6%) | 6 (1.9%) |
No response | 92 (11.9%) | 46 (13.4%) |
Dropout | 126 (16.4%) | 25 (8%) |
History of suicide attempts | ||
Yes | 250 (32.5%) | 96 (30.6%) |
No | 425 (55.2%) | 177 (56.4%) |
No response | 95 (12.3%) | 41 (13.3%) |
History of psychiatric hospitalizations | ||
Yes | 361 (46.9%) | 134 (42.7%) |
No | 310 (40.3%) | 139 (44.3%) |
No response | 99 (12.9%) | 41 (13.1%) |
Self-reported co-morbidities | ||
PTSD | 379 (49.2%) | 149 (47.5%) |
Substance Use Disorder | 75 (9.7%) | 26 (8.3%) |
Alcohol Use Disorder | 100 (13.0%) | 40 (12.7%) |
Anxiety Spectrum Disorders | 259 (33.6%) | 105 (33.4%) |
Obsessive Compulsive Disorder | 28 (3.6%) | 16 (5.1%) |
Bipolar Disorder | 90 (11.7%) | 33 (10.5%) |
Self-reported prior traumatic brain injury | ||
No | 304 (39.5%) | 150 (44.1%) |
Yes | 313 (40.6%) | 165 (48.5%) |
Unknown or Missing | 153 (19.9%) | 27 (7.4%) |
- a
-
Note that numbers may not equal 100% based on patient responses. “Entire Group” indicates those patients that received a baseline assessment and at least one TMS session, and “Adequate Dose” indicates those patients who received at least thirty sessions of TMS therapy.
- b
-
Includes: Asian Indian; American Indian; Native Alaskan; Pacific Islander; Middle Eastern.
Table 2. TMS approaches.
TMS Frequency and Target | n (%) |
---|---|
10 Hz left DLPFC | 595 (69.8%) |
5 Hz left DLPFC | 70 (8.2%) |
iTBS left DLPFC (600 pulses) | 59 (7.1%) |
1 Hz right DLPFC | 24 (2.8%) |
18 Hz dTMS | 67 (7.9%) |
1 Hz dTMS | 12 (1.4%) |
Other | 24 (2.8%) |
Abbreviations are as follows: Hz = hertz; DLPFC = dorsolateral prefrontal cortex; iTBS = intermittent theta burst; dTMS = deep transcranial magnetic stimulation.
FDA cleared TMS parameters included 10 Hz for Magstim, Neuronetics or Magventure systems for 4 s, 11–26 s intertrain interval (ITI), 3000 pulses per session; Brainsway devices using 18 Hz for 2 s, 20 s ITI, 1980 pulses; and intermittent theta burst delivering 50 Hz triplets at 5 Hz, 2 s stimulation with 8 s ITI, 600 pulses).
3.2. Clinical outcomes
3.2.1. MDD symptom change with TMS
When considering the entire cohort (i.e., baseline assessment and >1 TMS session), the sample included 770 veterans, and 126 (16.4%) were classified as dropping out (see below). Mean (SD) pretreatment PHQ9 score was 18.14 (5.18), indicating moderately severe MDD. After treatment, PHQ9 score was reduced to 11.02 (7.11), which was statistically significant (t(542)=25.996, p < .001). PHQ9 reduction was clinically meaningful at 7.12 (6.38) points (Fig. 1A) and reflected a large effect size (Cohen’s d)=1.12 (95% CI 1.04–1.21). Categorical outcomes included clinical response in 235 (30.5%) and remission in 118 (15.3%) patients. Regression co-varying for the presence of PTSD indicated no impact on PHQ9 change (F(1539)=0.065, p =.799).
Fig. 1. A Trajectory of MDD and PTSD symptoms across the entire group (n = 770).
Bars represent standard error of the mean. The Y-axis represents symptom scores, and X-axis describes week of TMS treatment.
Abbreviations: Pre-Tx, pre-treatment; Post-Tx, post-treatment; PHQ-9, 9-item patient health questionnaire; PCL-5, PTSD checklist for DSM-5.
B. Trajectory of MDD and PTSD symptoms in patients who received an adequate dose of at least 30 TMS sessions (n = 340).
Bars represent standard error of the mean. The Y-axis represents symptom scores, and X-axis describes week of TMS treatment.
Abbreviations: Pre-Tx, pre-treatment; Post-Tx, post-treatment; PHQ-9, 9-item patient health questionnaire; PCL-5, PTSD checklist for DSM-5.
Regarding PTSD outcomes in the entire cohort, 522 (67.8%) had threshold-level PTSD symptom severity at baseline. Pretreatment mean (SD) PCL-5 score was 54.22 (12.11), indicating moderate PTSD severity. After TMS, PCL-5 was reduced to 34.76 (19.81), which was statistically significant (t(364)=20.70, p < 0.001), and clinically meaningful with a reduction of 18.49 (17.05) points. This change also reflected a large effect size (Cohen’s d)=1.11 (95% CI 0.97–1.25). After TMS, 300 (57.4%) patients demonstrated a clinically meaningful reduction and 312 (59.8%) no longer met threshold criteria for probable PTSD – all statistically significant (p <.01). Changes in MDD and PTSD symptoms were highly correlated (r = 0.521, p <.001).
When considering MDD outcomes in patients who received an adequate TMS dose, 340 veterans remained (Fig. 1B). Pretreatment PHQ9 scores were 18.34 (5.24); after treatment, PHQ9 scores were 11.29 (7.08), which was statistically significant (t(339)=21.06, p <.001). PHQ9 scores improved 7.12 (6.21) points, which was clinically meaningful and reflected a large effect size (Cohen’s d = 1.15 (95% CI 1.00–1.30). Categorical outcomes included response in 149 (41.4%) and remission in 72 (20.0%) patients.
Regarding PTSD outcomes in patients who received an adequate dose, 245 (70%) had threshold level PTSD symptom severity at baseline. Pretreatment PCL-5 score was 52.67 (11.91), indicating moderate severity. After TMS, the mean PCL-5 score was 33.74 (18.87), which was statistically different (t(230)=17.85, p<.001). Improvements were clinically meaningful, with an 18.95 (16.14)-point reduction that reflected a large effect size (Cohen’s d = 1.20 (95% CI 1.01–1.38)). After TMS, 160 (65.3%) demonstrated a clinically meaningful reduction and 113 (46.1%) no longer met PTSD criteria. MDD and PTSD changes were highly correlated (r = 0.623, p<.001).
We performed several sensitivity analyses to explore important clinical questions. First, we found our results were not sensitive to the definition of adequate dose (i.e., 20 vs. 30 sessions). We also explored whether FDA-cleared parameters might yield superior outcomes on MDD or PTSD, and found no significant differences (Supplement 1).
3.2.2. Comparing MDD outcomes in veterans with probable PTSD versus non-PTSD
Because prior reports indicated mixed MDD outcomes in veterans with comorbid PTSD treated with TMS, we compared outcomes in veterans with versus without PTSD. When evaluating the entire cohort, MDD improved over time (F(1547)=207.654, p <.001) and the presence of clinically significant PTSD symptoms did not impact decreases in depressive symptoms (F(1547)=0.030, p >.862). Post-hoc analyses revealed individuals with PCL-5 scores >33 had higher PHQ-9 scores (i.e., PHQ9 scores of 19.64 (4.46) versus 14.48 (5.80) for those with versus those without threshold-level symptom severity; F(1659)=159.10, p <.001)(Fig. 2A). While PTSD did not impact the magnitude of reduction in depressive symptoms, MDD response rates were lower in PTSD patients (17.5% versus 26.7% for response rates in PTSD and non-PTSD, respectively; χ2(1,N = 558)=11.35, p=.001). Remission rates were statistically poorer in PTSD patients, although rates were nearly identical (11.2% versus 11.6% in PTSD and non-PTSD, respectively; χ2(1,N = 541)=27.18, p <.001).
Fig. 2. A. MDD outcomes in patients with and without threshold-level PTSD symptom severity in the entire cohort.
Key: Y-axis represents mean PHQ9 scores, and “PTSD” indicates threshold-level PTSD symptom severity. Error bars represent standard error of the mean.
B. MDD outcomes in patients with and without threshold-level PTSD symptom severity in patients who received an adequate dose of at least 30 TMS sessions.
Key: Y-axis represents mean PHQ9 scores, and “PTSD” indicates threshold-level PTSD symptom severity. Error bars represent standard error of the mean.
When comparing PTSD versus non-PTSD patients who received an adequate TMS dose, a similar pattern emerged; depressive symptoms improved over time (F(1373)=140.20, p <.010) and PTSD did not impact the magnitude of depression improvement (F(1373)=0.405, p >.525). Post-hoc analyses again indicated PTSD patients had higher baseline PHQ-9 scores (i.e., 19.70 (4.45) and 15.21 (5.57) for those with and without PTSD, respectively; F(1348)=63.86, p <.001)(Fig. 2B). Response rates for depression were lower in PTSD patients (26.6% versus 17.8% in PTSD and non-PTSD, respectively; χ2(1,N = 374)=12.96, p <.001). Remission rates were statistically lower in PTSD patients, although these rates were nearly identical (10.4% versus 11.0% for remission in PTSD and non-PTSD, respectively; χ2(1,N = 370)=17.35, p <.001).
3.3. Safety
TMS was safe, with significant adverse outcomes occurring in <1% of patients. Six reported exacerbations of significant underlying psychiatric symptoms during TMS, largely related to worsening depression or anxiety. Two had significant medical events unrelated to TMS. One veteran had a seizure while being treated with an H-coil system and treatment was discontinued. There were no contraindications or risk factors noted in the pretreatment evaluation of this patient. There were no reports of treatment-emergent mania. A total of n = 126 (16.4%) of the entire sample dropped out for any reason.
4. Discussion
This report describes a multisite retrospective cohort study of TMS for veterans with MDD. The majority of patients reported comorbid MDD and PTSD, and pretreatment symptom severity was at least moderate. TMS resulted in statistically significant and clinically meaningful symptom reduction in all measured domains. TMS was safe, with side effects (including one seizure) consistent with its known safety profile, and retention was high. To our knowledge, this is the largest study of TMS in veterans to date, and clearly demonstrates effectiveness.
MDD improvements were associated with large effect sizes (all Cohen’s ds >1.0), regardless of how one examined the data. When considering categorical outcomes, those who received an adequate dose (i.e., >30 sessions), MDD response rates were 41.4% and the remission rate was 20.0%. As expected, those who did not receive an adequate dose had lower response and remission rates (30.5% and 15.3%). These were slightly lower than prior naturalistic/uncontrolled studies of TMS for MDD, which likely reflects psychiatric and medical comorbidities in veterans (15–16). For example, Carpenter et al. (2021), reported response and remission rates of 56.4% and 28.7% in non-Veterans, and a recent a TMS registry report (non-Veterans) found response and remission rates up to 72% and 37%. For reference, all of these outcomes appear superior to acute remission rates in the STAR*D study, when considering comparably resistant patients (Sackeim 2016)).
Conversely, an earlier report of clinical outcomes of TMS for MDD in veterans found a similar response and remission rate (45% and 20%, respectively)(Kozel et al., 2017). In another study comparing standard TMS versus theta burst TMS for MDD (Blumberger et al., 2018), response and remission rates were 49% and 32%. Interestingly, remission rates observed in this cohort study were lower than in the controlled trial of TMS in veterans (20% here versus 37–40% in Yesavage et al. (2018)); the reason behind this discrepancy can only remain speculative.
These data demonstrate the importance of delivering an entire TMS treatment series. While overall magnitude of improvement (∼7 point reduction on the PHQ9; d =∼1.1) was comparable, response and remission rates were superior in patients that received >30 TMS sessions; this is not explained by differences in baseline severity Future studies will need to evaluate the relationship between the number of sessions required for optimal outcomes; however, since remission is the goal of antidepressant treatment, patients can be empirically recommended to receive six weeks of TMS.
Our cohort is notable as the majority reported significant comorbid PTSD. While some overlap is expected due to the nature of the rating scales (e.g., depressive questions and the mood/cognitive component of the PCL-5), over two thirds reported “threshold” level of PTSD severity. While structured clinical interviews (e.g., the Clinician Administered PTSD Scale (Weathers et al., 2013b)) were not performed, this comorbidity rate is high; as a point of reference, approximately 50% of patients in prior studies reported comorbid PTSD (Yesavage et al., 2018; Hernandez et al., 2020). In our estimation, this indicates the kind of VA patients most likely to be referred to TMS after prior treatment failures.
Observed PTSD outcomes are comparable to other studies of TMS for PTSD. PTSD improvements were statistically significant and clinically meaningful; of those patients with threshold-level PTSD symptoms at baseline, a near majority no longer met this criterion after TMS. This rate is similar to findings in smaller unblinded studies (e.g., Carpenter et al. 2018). In short, this study clearly demonstrates the effectiveness of TMS to reduce PTSD with comorbid MDD, and can be recommended for these patients.
Interestingly, the presence (or absence) of threshold-level PTSD symptoms did not impact the magnitude of observed MDD improvement (i.e., ∼7 points on the PHQ9). Yet, MDD response and remission rates in patients with comorbid PTSD were lower than those without PTSD. This appears to be related to the higher level of baseline MDD symptoms in those with PTSD, which would require a greater magnitude of symptom reduction to achieve response and remission. Further defining the relationship between PTSD and MDD domains in clinical care – such as whether one can be used to predict the other, or whether they can be considered independent – is an important area of further inquiry.
Perhaps remarkably, PTSD improved with 10 Hz stimulation to the left DLPFC, the traditional target for MDD. Whether there is an ideal TMS site for PTSD has received considerable attention, with studies using left-sided stimulation (Yesavage et al., 2018; Carpenter et al., 2018; Philip et al., 2016), right-sided stimulation (Kozel et al., 2019; Philip et al., 2019; Watts et al., 2012; Kozel et al., 2018), a mixture thereof (Ahmadizadeh and Rezai 2018; Leong et al., 2020; Boggio et al., 2010) or broader stimulation (Isserles et al., 2013, 2021); clear superiority of any approach has yet to be demonstrated (e.g., Philip et al. 2021). These results raise important questions as to whether there is an ideal TMS approach best suited for PTSD reduction. While future analyses of this database will compare various parameters, these results indicate left-sided stimulation can improve PTSD.
Limitations of this work are those inherent to cohort studies. Primarily, stimulation was unblinded and so the impact of placebo or nonspecific effects cannot be assessed. Outcomes relied heavily on self-reported clinical scales, and future examination of other scales and functional outcomes is anticipated. Patients were engaged in concurrent clinical care, and so we cannot identify the unique contribution of stimulation, and the effect of visit attendance may have induced an effect. Antidepressant resistance (i.e., how many failed prior trials) was not analyzed, to provide a broad estimate of real-world outcomes, and so we cannot comment upon whether or how antidepressant resistance was associated with clinical outcomes. We were also not able to precisely identify every reason for dropout. Patient demographics are consistent with VA studies, although many races, ethnicities and genders were represented. Future investigations will evaluate clinically important aspects of care, such as impact of varied parameters and medications (including changes thereof), TBI status, durability of effect, and effects of TMS on PTSD symptom domains. Future precision medicine studies (e.g., Williams et al. 2021), using this VA network will provide large-scale insight into clinical biomarkers of treatment response.
Caveats notwithstanding, this is the largest report of TMS in US military veterans and provides a clear indication of the safety and effectiveness of TMS. Greater exposure to TMS (i.e., more treatment sessions) yielded superior MDD outcomes, and TMS was broadly efficacious for PTSD. Comorbid PTSD was not clearly associated with poorer outcomes and as such, TMS can be recommended for this patient population.
Grant support
The VA Clinical TMS Program is supported by the VA Office of Mental Health and Suicide Prevention; VA provided clinical support for the program in terms of both equipment and workload for allocation of staff resources. Effort on this project was supported in part by the VA RR&D Center for Neurorestoration and Neurotechnology at the Providence VA (NSP), NIMH R01 MH120126 & VA I01 CX002088.
CRediT authorship contribution statement
Michelle R. Madore: Visualization, Investigation, Writing – original draft, Writing – review & editing. F. Andrew Kozel: Visualization, Investigation, Writing – original draft, Writing – review & editing. Leanne M. Williams: Writing – original draft, Writing – review & editing. L. Chauncey Green: Visualization, Writing – original draft. Mark S. George: Funding acquisition, Writing – original draft. Paul E. Holtzheimer: Writing – original draft, Writing – review & editing. Jerome A. Yesavage: Funding acquisition, Writing – review & editing. Noah S. Philip: Visualization, Investigation, Writing – original draft, Writing – review & editing.
Declaration of Competing Interest
In the last three years: LMW declares fees as Advisory Board Member for One Mind Psyberguide and as External Advisory Committee of the Laureate Institute for Brain Research, and US Pants. App. 10/034,645 and 15/820,338: Systems and methods for detecting complex networks in MRI image data, unrelated to this study. MSG has received equipment support for research from Magstim and Nexstim, and has conducted research at MUSC funded by Brainsway. PEH receives consulting fees from Abbott and royalties from UpToDate and Oxford University Press. NSP has received equipment support (through VA clinical trial contracts) from Wave Neuro. Other authors have no biomedical conflicts of interest to disclose.
The views expressed in this article are those of the authors and do not necessarily reflect the position or policy of the Department of Veterans Affairs or National Institutes of Health. The funders had no role in study design, data collection and analysis, decision to publish, or preparation of the manuscript. The data that support the findings of this study may be available from the corresponding author upon reasonable request, subject to Department of Veterans Affairs policies.
Acknowledgements
We thank all the VA clinical TMS sites and their patients. For full details of the program sites please see the associated website at the Sierra Pacific (VISN 21) MIRECC – MIRECC / CoE (va.gov). We would also like to thank Laura Lazzeroni, PhD for statistical support.
Leave a Reply
Want to join the discussion?Feel free to contribute!